Robotic surgery in orthopaedics: What does the future hold?
Authors: Edward T Davis and Fares S Haddad on behalf of the Robotic And Digital Assisted suRgery (RADAR) Advisory Group
Robotic surgery in orthopaedics: What does the future hold?
Edward Davis is a hip and knee arthroplasty consultant at the Royal Orthopaedic Hospital in Birmingham where he is the clinical lead for the arthroplasty department. He is an Honorary Professor at the University of Birmingham and is the orthopaedic curriculum lead at the University of Birmingham and Aston University. He is the Head of the undergraduate academy at the Royal Orthopaedic Hospital.
Fares Haddad is Professor of Orthopaedic and Sports Surgery and Divisional Clinical Director of Surgical Specialties at UCLH, and Director of the Institute of Sport, Exercise and Health (ISEH) at University College London. He is Medical Director of Orthopaedics, HCA UK and the Editor in Chief of the Bone and Joint Journal.
Interest in robotic assisted surgery in orthopaedics is rapidly increasing with the development of new systems and a generation of evidence on its safety and efficacy. The main applications have been in hip and knee arthroplasty1–3, with increasing interest in its usage in spinal surgery4,5. Robotic systems have been available since the 1990s but were slow to gain acceptance. The true definition of a robot is a “programmed actuated mechanism with a degree of autonomy to perform locomotion, manipulation or positioning”; the definition of autonomy being the “ability to perform intended tasks based on current state and sensing, without human intervention” (ISO standard 8373:2021)6. Therefore, many of the systems in current use do not fulfil the true definition of a robot and are referred to as robotically assisted surgical devices (RAS) by the FDA7. These devices form part of the wider group that are categorised as computer assisted surgical systems that can be used for pre-operative planning, surgical navigation and assisting surgical procedures.
One of the first orthopaedic robots was the ROBODOC system (Curexo Technology, Fremont, CA, USA), which was a fully autonomous device with the surgeon able to plan the bone preparation and the robot executing this without any active input from the surgeon. The CASPAR robot (Ortho- Maquet/URS, Schwerin, Germany) was also available at a similar time performing in a similar autonomous manner to that of the ROBODOC. These early systems were probably slow to gain widespread adoption, due to the complexities of use and the inability of the robot to distinguish bone from soft tissue. Concerns over the fully autonomous nature of these robots led to the advent of robotic assisted surgery and the development of the ACROBOT (Acrobot Ltd, London, UK) and the RIO Robotic Arm Interactive Orthopedic System (MAKO Surgical Corp., Lauderdale, FL, USA). The number of surgical robots now available for hip and knee arthroplasty has rapidly expanded.
These different systems provide different functionalities in hip and knee arthroplasty. Some provide an active robotic device that positions a cutting guide in the correct orientation, to then allow the surgeon to make the bone cuts using a standard surgical saw, whilst other systems may provide a cutting burr in a small handheld device that has the ability to retract when it is not positioned in an area that requires bone resection. Other devices, such as the MAKO and VELYS (Johnson and Johnson) hold the saw and guide the bone cuts. The different robotic assisted systems use pre-operative CT scans, pre-operative radiographs or use the acquisition of intra-operative anatomical landmarks to provide the three-dimensional plan that guides the bone resections. The levels of automation, active control, cutting tool integration, boundary or soft tissue protection and pre-operative imaging between the different systems, still need to be fully evaluated. The level of evidence for some systems is far greater than for others as summarised in the recent BOA / RCSEng / RCSEd documents, available on the BOA website at www.boa.ac.uk/robotics.
The future of robotic assisted surgery hardware
There has been a recent revolution in the miniaturisation of many technologies, noticeably in mobile telephone capabilities. Within other areas of surgery there is currently a shift to smaller robotic devices, such as the use of natural orifice robotic devices that can undertake procedures within the body using natural orifices as the access point8. Within orthopaedics, the requirements to hold cutting guides or surgical tools with stability, has led to robotic assisted systems being large and cumbersome devices. As technology progresses, we anticipate these systems becoming smaller and more agile. The move away from traditional instruments to the use of burrs, lasers or piezotomes9,10 (such as in figure 1), may allow a reduction in size requirements for these devices with a transition to what can be termed smart instruments11. The miniaturisation of robotic assisted surgery may bring the added benefits of costs savings on the use of standard instrumentation and disposables12. The ability to use these technologies on revision knee replacement has been explored and has the potential to significantly reduce the need for mechanical instruments and guides thereby adding to cost and efficiency savings13.
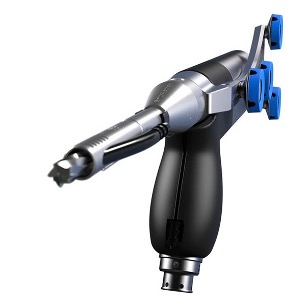
Figure 1: The robotic burr, example of a smaller 'smart tool'.
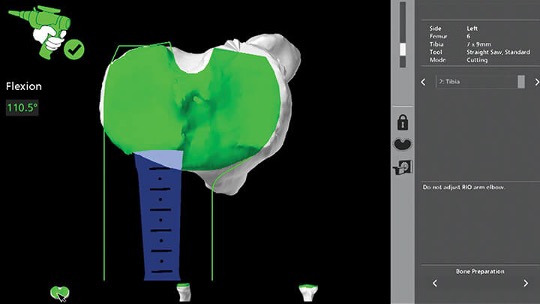
Figure 2: Screen shot of the robotic system showing the boundary control protecting the soft tissue envelope during tibial resection.
One of the main concerns of the fully autonomous robots was the protection of soft tissues. The addition of haptic or ‘hard stop’ boundaries during the bone preparation that is found within the CT Based Mako system (as seen in figure 2), and the imageless CORI system is an area that is certain to expand and may be one of the fundamental benefits of this type of technology14.
Improvements in the ability of the systems to identify soft tissue so that they can be protected, is an important area and in time may allow us to revisit fully autonomous systems. Improvements in the development of intra-articular sensors that can be used particularly during knee replacement to measure soft tissue tensions and optimise ligament balancing, is variable between the different systems and is currently under investigation into how these data can be interpreted and acted on, to provide better outcomes. Again, the ability to miniaturise sensors is of benefit and these types of soft tissue balancers are a key area that will improve the use of robotic assisted surgery to optimise component orientation in a personalised way15,16.
Most of the current robotically assisted surgery platforms rely on infrared trackers, which are fixed to the bone and require an infrared emitter and a camera to have direct line of sight. This often presents logistical problems of organising the operating staff and equipment but also in the added procedure of fixing the arrays to the bone17. Current developments are utilising the rapid expansion in the field of machine vision, to try to eliminate the requirement for fixed trackers placed in the bone and utilise optical cameras to identify the exposed bone surfaces18. This, combined with the improvements in miniaturisation of cameras, would eliminate the need to have a single fixed camera, opening up the possibility of using multiple cameras so that if one is obstructed then data can still be acquired. Ideally, the camera could be in a position to track the surgeon’s line of sight19.
Developments in augmented reality systems will also play a part in future developments, helping the surgeon visualise the anatomy and implant positioning during the planning stages20,21. Augmented reality headsets are now more ergonomic and will provide surgeons with data and information within the surgical field. However, there will need to be a careful balance between providing important and meaningful data at the appropriate time vs simply distracting and overloading the surgeon with information22. There have been very significant advances in the field of virtual reality23, which simulates the operation to either enable surgeons to learn the procedure or for pre-performance practise24, which is known to be beneficial when completing technical tasks. Robotically assisted surgical systems often disrupt the standard dynamics within an operating theatre with potential detrimental as well as beneficial effects on team working and the non-technical aspects of surgical procedures25. Virtual reality has the potential to help simulate these environments and to train staff and teams on the integration of technologies into the operating theatre environment.
Software and artificial intelligence/machine learning
One of the most rapidly advancing fields is in the software for robotic assisted systems. This provides huge opportunities but also complexities in analysing the outcomes of different systems, especially when multiple versions have evolved since the system was first evaluated. The ability for precise tracking and the wealth of data that these systems collect is leading to a better understanding of optimising component orientation in knee replacement and will certainly expand to other areas of orthopaedics. The ability to track the surgical tools has been shown in other disciplines such as urology to even predict post-operative outcomes and discriminate on surgeon performance26. Many current systems still require significant human input from technicians in the segmentation and planning stages of robot assisted surgery, which is time consuming and costly. Many of these human contacts could be eliminated using analytics based on artificial intelligence (AI). Many systems still require complex surgical decision-making in interpreting data provided by the robotic systems, leading to possible cognitive overload with the potential for component malpositioning or errors. AI systems and data analytics provide the benefit of being able to analyse previous data sets and provide optimised component orientation plans or provide prompts (nudges) to guide surgeons to the most appropriate position. Advanced analytics such as AI and machine learning can easily absorb and analyse these very large data sets; combining these with the electronic patient record produces a personalised plan based on validated data sets. AI and machine learning can already predict complications and outcomes following joint replacement27-29 and its integration into robotic systems will potentially enhance pathways and outcomes.
However, AI and machine learning bring concerns with data governance and data protection. Concerns on sharing anonymised large data sets, which may allow patient identification due to the granularity of the data will require changes in data protection law and possibly more punitive charges if individuals or organisations actively reidentify patients from anonymised datasets30.
The future of robotic assisted surgery workforce and education
One of the most controversial areas within the development of robotic assisted surgery in orthopaedics has been the implication on training and education31. Concern regarding the ability of trainees to gain access to robotic systems for training and more importantly for performing the surgery (rather than assisting) presents many challenges. Equality of access to these systems for both patients and trainees needs to be resolved32. During the adoption phases, trainees are often displaced while the consultant surgeon becomes proficient at using these systems and therefore not only access to robotic systems but access to robotic systems in centres with experienced users is required. As widespread adoption occurs, these issues will become less pertinent but in the transition phase, solutions will be needed. During the transition phase it will be important that training surgeons gain access to robotic systems but also that their training is balanced with an understanding how to use conventional instrumentation. As robotic systems become the standard of care then the necessity to use standard instrumentations will be less, but there will still be the requirement to understand how standard instrumentations work in the event of a technical failure.
Assisted technologies such as navigation and robotics have the potential to accelerate education in surgical specialties and improve the understanding of surgical techniques such as ligament balancing in knee replacements and component optimisation in both hip and knee replacement33, as figure 3 shows with a wealth of information to plan surgery. Future work will improve the training methodologies that are used to educate surgeons in robotic technologies. Different educational methodologies may be required. Within the field of robotically assisted prostatectomy, data taken from the robotic systems can help delineate the skill set of different surgeons34, who can then focus training needs on specific aspects of surgical performance.
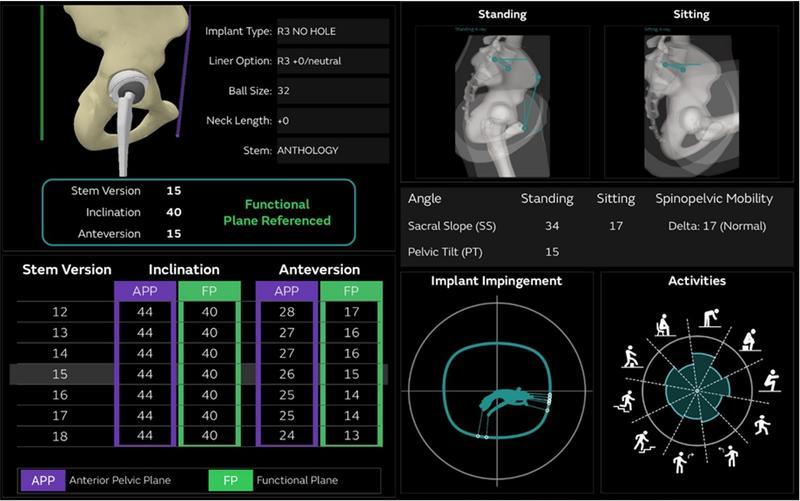
Figure 3: Personalising component orientation using the hip modeler showing impingement in activities of daily living.
Implications for surgical workforce planning may also be heavily influenced by the development and implementation of these technologies. Currently, all of the systems require significant surgeon involvement and surgical skill and therefore the surgeon must be present during the procedure. As the systems advance and move towards more intuitive designs encompassing decision-making, component position optimisation, soft tissue protection and automation, then the requirement for the surgeon to be present throughout the surgery may reduce. This may allow the surgeon to be responsible for running multiple operating theatres with more reliance on allied health professions and technicians for certain elements of the procedure. This will enable the surgeons to be more focused on dealing with complex anatomy or scenarios where there may be ambiguity in the optimisation of component orientation or dealing with complications. This area brings with it huge controversy and will be difficult to navigate. Parallels with the acceptance of fully autonomous cars and the presence of human pilots being required despite aircraft being more than capable of automated flight have shown that this area is highly controversial and often takes much longer to adopt even when technology is available to undertake the tasks.
The future of robotic assisted surgery legal and ethical implications
The development and adoption of robotic assisted surgery is intrinsically linked with big data and the requirements of sharing data across different providers and regions as well as between industry and the healthcare sector. The legal and ethical implications of this type of data sharing must be addressed and be transparent to patients35. Many of these issues are already being addressed in other sectors, such as within the automotive industry who are leading on the issues of privacy, as automated cars continually recording their surroundings. As discussed earlier, there may be a requirement to move forward with more punitive charges for individuals or companies who misuse big data in an effort to allow more open data sharing and a more rapid development of these systems. Concerns over the validity of AI algorithms on minority groups will need to be addressed and transparency on the training data sets used in the development of these algorithms will need to be robust.
Patients perception of robotic assisted surgery will also need to be more fully explored as we move to more automated technology and this will certainly dictate the pace of change depending on patient confidence in this technology. The accountability and liability if complications occur will also be a challenging area particularly as the technology takes on more decision-making functionality and as the systems progress to become more autonomous.
Research and evidence generation
The ability to demonstrate the safety and efficacy of these systems will be fundamental to the pace of adoption. Health economic research will also be critical with the increasing challenges that healthcare systems are put under in trying to reduce costs and improve care. Conducting research into rapidly evolving technologies has always been challenging particularly where software iterations are continually changing. Clinical studies and real world evidence will be important in the development and adoption of this technology with large randomised controlled trials already being conducted. One of the main challenges will be the classification of different systems and different software algorithms that may provide very different benefits and risks to patients.
Conclusion
Robotic assisted surgery in orthopaedics is a rapidly evolving area and brings with it the hope of improving patients’ outcomes with particular focus on consistency and quality of surgical outcome. As systems evolve and develop it is vital that we maintain safety whilst also being cognisant of the health economic challenges that we face. Technology is rapidly evolving in all areas of our lives and will certainly be part of surgical practise in the future. It is vital that the adoption is undertaken supported by evidence-based medicine and addresses the potential changes in the way that we provide education and training in this area to both surgeons in training and established consultants. These systems potentially allow a more personalised approach to surgery and these factors need to be considered when looking at evidence generated on their efficacy. The current RACER trials and other level 1 studies will help inform and possibly dictate the pace of change with respect to hip and knee arthroplasty and are eagerly awaited14,36-38.
References
- Vermue H, Batailler C, Monk P, Haddad F, Luyckx T, Lustig S. The evolution of robotic systems for total knee arthroplasty, each system must be assessed for its own value: a systematic review of clinical evidence and meta-analysis. Arch Orthop Trauma Surg. 2023;143(6):3369-81.
- Riantho A, Butarbutar JCP, Fidiasrianto K, Elson E, Irvan I, Haryono H, Prasetio JN. Radiographic Outcomes of Robot-Assisted Versus Conventional Total Knee Arthroplasty: A Systematic Review and Meta-Analysis of Randomized Clinical Trials. JBJS Open Access. 2023;8(2):e23.00010.
- Ma M, Song P, Zhang S, Kong X, Chai W. Does robot-assisted surgery reduce leg length discrepancy in total hip replacement? Robot-assisted posterior approach versus direct anterior approach and manual posterior approach: a propensity score-matching study. J Orthop Surg Res. 2023;18(1):445.
- Lopez IB, Benzakour A, Mavrogenis A, Benzakour T, Ahmad A, Lemée JM. Robotics in spine surgery: systematic review of literature. Int Orthop. 2023;47(2):447-56.
- Perfetti DC, Kisinde S, Rogers-LaVanne MP, Satin AM, Lieberman IH. Robotic Spine Surgery: Past, Present, and Future. Spine (Phila Pa 1976). 2022;47(13):909-21.
- ISO 8373:2021 - Robotics Vocabulary. Available at: www.iso.org/standard/75539.html.
- U.S. Food and Drug Administration (FDA). Computer-Assisted Surgical Systems. Available at: www.fda.gov/medical-devices/surgery-devices/computer-assisted-surgical-systems.
- Simsek C, Kaan HL, Aihara H. Future Directions for Robotic Endoscopy–Artificial Intelligence (AI), Three-Dimensional (3D) Imaging, and Natural Orifice Transluminal Endoscopic Surgery (NOTES). Tech Innov Gastrointest Endosc. 2023;25(1):95-101.
- Zhang Y, Wang C, Zhou S, Jiang W, Liu Z, Xu L. A Comparison Review on Orthopedic Surgery Using Piezosurgery and Conventional Tools. Procedia CIRP. 2017;65:99-104.
- Holzinger D, Ureel M, Wilken T, Müller AA, Schicho K, Millesi G, Juergens P. First-in-man application of a cold ablation robot guided laser osteotome in midface osteotomies. J Craniomaxillofac Surg. 2021;49(7):531-7.
- Bollars P, Meshram P, Al Thani S, Schotanus MGM, Albelooshi A. Achieving functional alignment in total knee arthroplasty: early experience using a second-generation imageless semi-autonomous handheld robotic sculpting system. Int Orthop. 2023;47(2):585-93.
- Christen B, Tanner L, Ettinger M, Bonnin MP, Koch PP, Calliess T. Comparative Cost Analysis of Four Different Computer-Assisted Technologies to Implant a Total Knee Arthroplasty over Conventional Instrumentation. J Pers Med. 2022;12(2):184.
- Wu XD, Zhou Y, Shao H, Yang D, Guo SJ, Huang W. Robotic-assisted revision total joint arthroplasty: a state-of-the-art scoping review. EFORT Open Rev. 2023;8(1):18-25.
- Kayani B, Tahmassebi J, Ayuob A, Konan S, Oussedik S, Haddad FS. A prospective randomized controlled trial comparing the systemic inflammatory response in conventional jig-based total knee arthroplasty versus robotic-arm assisted total knee arthroplasty. Bone Joint J. 2021;103-B(1):113-22.
- Sharma AK, Plaskos C, Shalhoub S, Lawrence D, Vigdorchik JM, Lawrence JM. Ligament Tension and Balance before and after Robotic-Assisted Total Knee Arthroplasty - Dynamic Changes with Increasing Applied Force. J Knee Surg. 2024;37(2):128-34.
- Chang JS, Kayani B, Wallace C, Haddad FS. Functional alignment achieves soft-tissue balance in total knee arthroplasty as measured with quantitative sensor-guided technology. Bone Joint J. 2021;103-B(3):507-14.
- Thomas TL, Goh GS, Nguyen MK, Lonner JH. Pin-Related Complications in Computer Navigated and Robotic-Assisted Knee Arthroplasty: A Systematic Review. J Arthroplasty. 2022;37(11):2291-2307.e2.
- Félix I, Raposo C, Antunes M, Rodrigues P, Barreto JP Towards markerless computer-aided surgery combining deep segmentation and geometric pose estimation: application in total knee arthroplasty. Comput Methods Biomech Biomed Eng Imaging Vis. 2021;9:271-8.
- Hu X, Baena FRY, Cutolo F. Head-Mounted Augmented Reality Platform for Markerless Orthopaedic Navigation. IEEE J Biomed Health Inform. 2022;26(2):910-21
- Lex JR, Koucheki R, Toor J, Backstein DJ. Clinical applications of augmented reality in orthopaedic surgery: a comprehensive narrative review. Int Orthop. 2023;47(2):375-91.
- Iqbal, H., Tatti, F. & Rodriguez y Baena, F. Augmented reality in robotic assisted orthopaedic surgery: A pilot study. J Biomed Inform 120, 103841 (2021).
- Dias, R. D. et al. Intelligent interruption management system to enhance safety and performance in complex surgical and robotic procedures. Lecture Notes in Computer Science (including subseries Lecture Notes in Artificial Intelligence and Lecture Notes in Bioinformatics) 11041 LNCS, 62–68 (2018).
- Hasan, L. K. et al. Virtual Reality in Orthopedic Surgery Training. (2021) doi:10.2147/AMEP.S321885.
- Cragg, J. et al. Surgical cognitive simulation improves real-world surgical performance: randomized study. BJS Open 5, (2021).
- Manuguerra, A. et al. Non-technical skills in robotic surgery and impact on near-miss events: a multi-center study Non-technical skills in robotic surgery and impact on near-miss events: a multi-center study Non-technical skills in robotic surgery and impact on near-miss events: a multi-center study. Surg Endosc 2021,.
- Hung, A. J. et al. A deep‐learning model using automated performance metrics and clinical features to predict urinary continence recovery after robot‐assisted radical prostatectomy. BJU Int 124, 487–495 (2019).
- Goltz, D. E. et al. A Novel Risk Calculator Predicts 90-Day Readmission Following Total Joint Arthroplasty. J Bone Joint Surg Am 101, 547–556 (2019).
- Farooq, H., Deckard, E. R., Ziemba-Davis, M., Madsen, A. & Meneghini, R. M. Predictors of Patient Satisfaction Following Primary Total Knee Arthroplasty: Results from a Traditional Statistical Model and a Machine Learning Algorithm. J Arthroplasty 35, 3123–3130 (2020).
- Pua, Y. H. et al. Machine learning methods are comparable to logistic regression techniques in predicting severe walking limitation following total knee arthroplasty. Knee Surg Sports Traumatol Arthrosc 28, 3207–3216 (2019).
- Aggarwal, R., Farag, S., Martin, G., Ashrafian, H. & Darzi, A. Patient Perceptions on Data Sharing and Applying Artificial Intelligence to Health Care Data: Cross-sectional Survey. J Med Internet Res 23, (2021).
- Haddad, F. S. & Horriat, S. Robotic and other enhanced technologies: Are we prepared for such innovation? Bone and Joint Journal 101-B, 1469–1471 (2019).
- Fleming, C. A. et al. Surgical trainee experience and opinion of robotic surgery in surgical training and vision for the future: a snapshot study of pan-specialty surgical trainees. J Robot Surg 16, 1073–1082 (2021).
- Cobb, J. P., Kannan, V., Brust, K. & Thevendran, G. Navigation Reduces the Learning Curve in Resurfacing Total Hip Arthroplasty. Clin Orthop Relat Res 463, 90–97 (2007).
- Hung, A. J. et al. Experts vs super-experts: differences in automated performance metrics and clinical outcomes for robot-assisted radical prostatectomy. BJU Int 123, 861–868 (2019).
- Naik, N. et al. Legal and Ethical Consideration in Artificial Intelligence in Healthcare: Who Takes Responsibility? Front Surg 9, 862322–862322 (2022).
- Griffin, J. et al. Robotic Arthroplasty Clinical and cost Effectiveness Randomised controlled trial (RACER-knee): a study protocol. BMJ Open 13, e068255 (2023).
- Kayani, B., Konan, S., Tahmassebi, J., Ayuob, A. & Haddad, F. S. Computerised tomography-based planning with conventional total hip arthroplasty versus robotic-arm assisted total hip arthroplasty: Study protocol for a prospective randomised controlled trial. Trials 21, 1–10 (2020).
- Kayani, B. et al. Robotic-arm assisted medial unicondylar knee arthroplasty versus jig-based unicompartmental knee arthroplasty with navigation control: Study protocol for a prospective randomised controlled trial. Trials 21, 1–11 (2020).